The
cyclic nature of elementary particles
The
Forgotten Lesson from Quantum World
Donatello
Dolce

The assumption of an intrinsically cyclic nature of
elementary systems represents a fundamental principle to
address in a natural way important open (and sometime
“forgotten over the decades”) questions in physics and
beyond. In fact intrinsic periodicity for elementary systems
provides a concrete formalization of the undulatory nature of
elementary particles at the base of the ordinary wave-particle
duality. This also means that every elementary particle can be
represented as a reference clock and its interaction described
as modulation of periodicity, similarly to general
relativity. The resulting theory is extremely intuitive and
natural, nonetheless it must be noticed that it has been
formulated in a rigorous mathematically way. For instance, in
recent publication in leading reviewed journals of theoretical
physics [1,4], it has been proved that the theory formally
reproduces the most fundamental aspects of the modern Quantum
Field Theory. Here we will presents some basic aspects of this
innovative interpretation of the quantum realm.
|
I. The ‘incomplete revolution’ of
quantum physics.
Quantum mechanics (QM) has been
defined the “incomplete revolution”. In fact it is an
“axiomatic” theory, i.e. based on purely mathematical axioms
whose physical meaning, after more than a century, is still
debated. From a formal point of view QM works in surprising
agreement with our experimental observations. This means that its
mathematical formulation must be considered to be correct. But
over the decades it has left unsolved a consistent number of
paradoxes and questions. The elusive nature of the quantum world
has been pointed out by the most eminent minds of quantum physics.
Einstein addressed the indeterministic nature of QM by saying
“God doesn’t play dice?”. Feynman, who is the father of the
most powerful and modern formulation of QM, said “I think I can
safely say that nobody understands QM”.
The most
problematic aspects arise when QM is combined to relativity. In
this case the difficulties are also computational and
mathematical. In fact observables in particle physics are
calculated through techniques that involves cancellation, a.k.a.
renormalization, of infinities (e.g. loop diagrams). These
calculation are so complex that two of the fathers of
renormalization theory, Prof. G. ‘t Hooft and Prof. F. Wilzcek,
both important Nobel laureates, are actively working on
foundations of QM --- in models that for some aspects are
related to the present proposal. To add a single digit in the
precision of these calculations nowadays are necessary brute-force
calculations with long simulations in extremely expensive
supercomputers. Indeed, besides the purely conceptual
interest, the open questions of QM affect directly most of the
ongoing research areas of modern physics. For instance the idea of
the Higgs boson (a.k.a. God’s particle) originates from
superconductivity (Cooper pair), that is a (not completely
understood after a century) quantum phenomenon. The problematics
of the Higgs sector are essentially of quantum nature (e.g. vacuum
stability, radiative corrections, hierarchy problem,
unitarization).
Another example is that, in General
Relativity (GR), gravitational interaction has a well-established
geometrical space-time meaning. On the other hand gauge
interactions, investigated by LHC and representing the other three
fundamental interactions of nature, are based on quantum
symmetries. Despite Weyl’s [43] and Wheeler’s original
attempts to interpret these so-called gauge symmetries, their
geometrical space-time meaning remains obscure and their existence
must be postulate mathematically.
Furthermore the situation
is even worse when QM is combined to GR. In fact, theories like
quantum gravity or string theory are becoming more and more
speculative and unnatural. One of the most important outcomes of
these theories, the so-called gauge/gravity duality or AdS/CFT
correspondence [42], remains after more than a decade an unproved
conjecture, a.k.a. Maldacena’s conjecture. A similar
speculative tendency can also be observed in High Energy Physics
(HEP). Data coming from the new experiments are in fact excluding
or strongly constraining the most popular and investigated models
of new physics beyond the standard model, including minimal models
of supersymmetry and extra dimensions. Of course it is always
possible to extend these models introducing new sectors [7] (i.e.
more untested hypothesis), but this inevitably involves new
parameters. As a result the constraints on the model can be
relaxed but at the same time this decreases the predictivity,
falsifiability and naturalness of the models. LHC has only left a
tiny allowed region for the Higgs boson (mostly between
122-128 GeV, for comparison the original theoretical allowed
region was about 0-500 GeV, whereas the indirect from indirect
observation was about 40-170 GeV). Nevertheless this remaining
region is problematic even for the SM itself. For instance this
implies either that gauge interactions are practically decoupled
from gravity or that the vacuum of the universe is unstable.
On the other hand, if the Higgs boson will not be observed,
radically new quantum mechanisms for the generation of the mass of
ordinary matter are required, see for instance
Higgsless/Composite-Higgs models [6,5] or superconductivity
[1,32,33]. Whether the Higgs boson will be discover or not,
radically new ideas in particle physics will be required (I would
like to mention that even WIMPs, the main candidate for
dark-matter, is almost excluded by recent experiments).
As
noticed by G. ‘t Hooft and many other well-regarded physicists,
the new experimental constraints will eventually give rise to a
renewed interest on foundations of physics. In particle
physics we typically assume that there is something that we don’t
see for some experimental reason. Nevertheless data and anomalies
coming from new experiments suggest us that the correct attitude
to solve the problems of modern physics should be rather to think
that there is something that we do not fully understand about the
quantum realm.
II. Digging into the history of physics
As in a Pandora box, the simple single assumption of intrinsic
periodicity of elementary systems, introduced by de Broglie in
terms of “periodic phenomena”, unlocks in a natural way the
important unsolved (and sometimes “forgotten over the decades”)
problems of physics mentioned above, according to
[1-4,8-9,30-33]. The approach is so innovative, original and
exciting but, at the same time it has deep roots the history of
physics. It worth to use the report of the Ann. Phys. referee of
my recent paper [1] to introduce the issue in the most objective
way.
The referee, in Feb 2012 wrote: “In this paper the
author revisits an old question due to de Broglie about the
physics of the "periodic phenomenon" which is implicit
in the standard quantum mechanical treatment of (relativistic)
particles. Experiment tells us that a particle of mass M, at rest,
is to be associated with a temporal period of size T=h/M c^2 [h is
the Planck constant]. When the particle moves [with momentum p], M
gets replaced by the relativistic energy, and a corresponding
spatial period l=h/p emerges. The natural question,
unexplained by standard quantum mechanics, is about the physical
nature of the "something" which actually carries these
periodicities. While in the ordinary theory free particle wave
functions are designed by hand so as to realize these
periodicities, no physical system is associated to them, in the
way a pressure field is associated to a sound wave, for instance;
thus questions like "what is the clock that ticks with period
h/M " remained unanswered. In several earlier papers,
reviewed and extended in the present one, the author [D. Dolce]
has made a concrete proposal for a physical system exhibiting de
Broglie's periodic phenomenon, namely a classical Klein-Gordon
field (for spinless particles) which is subject to periodic
boundary conditions in space and time. He then argues that
standard first quantized semiclassical quantum theory is recovered
in this setting, see sect. 5 of the present paper for those
arguments. The paper is certainly important in that it
correctly pinpoints an open issue of the standard theory which is
of central importance. To some extent it had been forgotten over
the decades, but it is by no means clear that, equipped with
modern concepts and technology, it is necessarily impossible to
make progress here. The solution proposed by the author appears
natural and plausible as the Klein-Gordon field he employs also
makes its appearance in standard SECOND quantization, while the
formalism presented is basically a substitute for semiclassical
first quantization. This approach is certainly very inspiring and
intriguing. The new results in the present paper concern the
interpretation of the electromagnetic interaction (and non-abelian
generalizations) in this framework. The author proposes to
interpret the vector potential A_{\mu}(x) as defining an
infinitesimal local change of the periods T and l
[space-time periodicities]; this is motivated by the rule of
minimal substitution p_\mu -> p_\mu +e A_\mu [gauge
interaction], implying T^\mu \rightarrow T^\mu +e A^\mu /h for the
reciprocal periods [modulation of periodicity]. He describes a
reinterpretation of gauge transformations as geometrical
transformations acting on the space of periodic Klein-Gordon
fields with all possible periods”.
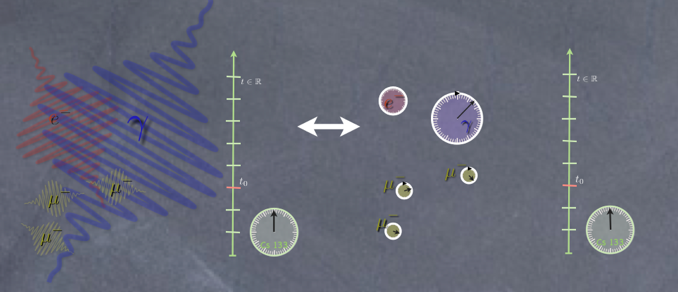
In
the left side of the (Fig.1a) shows the ordinary description of
a system of elementary particles (e.g. a photon, an electron
and three muons) in terms of waves. Such an description must be
generalized to QFT as relativistic corrections are considered.
In the right side (Fig.2a) the same system of particles in
terms of original de Broglie “periodic phenomena” which in
1924 gave rise the wave-particle duality and thus to the modern
undulatory description of QM. The de Broglie “periodic
phenomena” can be represented as clocks, the so-called de
Broglie “internal clocks” of the elementary particles. In
fact “By a clock we understand anything characterized by a
phenomenon passing periodically through identical phases”
[Einstein,1910]. The periodicity of these clock can vary
from 0 to infinity in the case of the photon. Matter particles
have tyoically an incredibly fast time periodicity. For the
electron it is faster that 10^-21 s whereas for the muon is
faster than 10^-23 s. In both figures it is also
represented the Cs-133 atominc clock whose periodicity is of
the order of 10^-10 s. The “ticks” of the Cs-133 clock is
used to defined the external time arrow. Every generic instant
of time t_0 can be characterized by an combinations of the
ticks of the de Briglie “internal clocks” of the particles
constituting the system as in a calendar or in a stopwatch.
The scale difference between the “ticks” of a Cs-133 clock
and of the internal clock of an electron is of the order of the
difference between the age of the universe and a solar year.
|
As well known, in the so-called
“old” formulation of QM, to every elementary particle is
associated a wave. This famous wave-particle duality is shown in
fig.1a. A wave is a phenomenon which has recurrences in time
(periodicity) and space (wavelength). Nearly 90 years ago de
Broglie noticed that, in the microscopic world, periodicity and
wavelength, T and l respectively, of a relativistic wave can
be used to describe the energy momentum, E and p, of the particle
through the Planck constant h. That is, E= h / T and p = h/ l
(these relations are often written in terms of time frequency and
spatial wavenumber). The undulatory nature of elementary particles
is at the base of the modern description of relativistic QM
and it is confirmed by many experiments. However, if
we want to include Special Relativity in QM it is necessary to
introduce Quantum Field Theory (QFT), i.e. an evolution of the
wave-particle duality based on more complicated and axiomatic
quantizations prescriptions, such as second quantization and
Feynman Path Integral (FPI).
Nevertheless in physics the most groundbreaking ideas are the
simple ones. To see how the simple assumption of intrinsic
periodicity can address the central questions listed above we must
reconsider the concept of elementary particle and of wave-particle
duality as originally introduced by de Broglie in 1922: “we
proceed with the assumption of the existence of a certain
“periodic phenomenon” of a yet to be determined character,
which is to be attributed to each and every isolated energy parcel
[elementary particle]” [de Broglie:1924,1924]. As the referee
noticed, the theory that I am going to introduce is a (literal)
realization of this yet to be determined de Broglie “periodic
phenomenon”, a.k.a. de Broglie “internal clock”. Similarly
to the wave-particle duality, the de Broglie temporal-spatial
periodicity describes the energy-momentum of the particle through
the Planck constant h. For the sake of simplicity here we will
consider only time periodicity T and energy E. Thus to the time
periodicity T there is associated a fundamental energy E=h/T. The
only assumption that we need is that every elementary isolated
particle has intrinsic de Broglie periodicity. This
assumption arises naturally as we combine the wave-particle
duality to Newton’s law of inertia. For instance, in
classical-relativistic mechanics an isolated elementary particle
has constant energy E, so that the corresponding temporal
periodicities of the de Broglie periodic phenomenon are
persistent, i.e. T is constant. We can for instance imagine
a pendulum (to avoid the use of the gravitational external force
we may consider a system of two masses and a spring) in the
vacuum. If no interaction or friction affect the pendulum, the
physics laws says that it will continue to oscillate forever with
the same periodicity fixed by the energy initially given to it. It
is important to bear in mind that, according to de Broglie, the
energy E and the periodicity T are “two faces of the same
coin”. This means that an assumption of intrinsic
periodicity is fully consistent with relativity as long as the
relativistic modulations of periodicity associated to relativistic
variations of kinematical state are considered. In fact we will
describe interaction as modulation of periodicity as for
gravitational interaction. Since our world is composed by
elementary particles, and elementary particles are intrinsically
periodic phenomena, it is natural to assume that reality can be
described iin terms of elementary cycles. As we will see below
this is the teaching of QM.
As Galileo taught us with
the pendulum experiment in the Pisa dome, time can only be defined
by counting the number of periods of a phenomenon which is
supposed to be periodic. The persistence of periodicity guaranties
that the unit of time does not vary. The modern definition of time
is based on the same principle: “A second is the duration of
9,192,631,770 periods of the radiation corresponding to [...] the
Cs 133 atom.”. The importance of the assumption of intrinsic
time periodicity is also present in Einstein’s definition of
relativistic clock: “By a clock we understand anything
characterized by a phenomenon passing periodically through
identical phases so that we must assume, by the principle of
sufficient reason, that all that happens in a given period
is identical with all that happens in an arbitrary period”
[Einstein, 1910]. Therefore, under the assumption of intrinsic
periodic periodicity, every elementary isolated particle can be
regarded as a reference clock, the so-called de Broglie “internal
clock” [38,39] (an isolated particle has constant energy E and
therefore it has persistent time periodicity T, similarly to a
inertial pendulum in the vacuum). In fig.1b, in fact, we
show the same systems of elementary particles of fig.1 (e.g. one
photon, one electron a three muons) described in terms of de
Broglie “internal clocks”. By assuming that an
elementary particle is an intrinsically periodic phenomenon we are
enforcing of the undulatory nature of elementary particles of
ordinary QM, and at the same time we are also enforcing the local
nature of relativistic time. In fact fig.1b also shows the arrow
of time defined in terms of the periods of the Cs atomic clock
(green). Every instant in time is characterized by a different
combination of the phases of the internal clocks constituting the
system under observation. This is what we do every day when we fix
events in time by combining phases of time periods that we call
years, months, days, hours, minutes, seconds, and so on.
As well known, a system of periodic phenomena is ergodic
(i.e. quasi-periodic evolution, such as that of the combination of
the rotation and the revolution of the earth, which causes the
addition of a day every four months and further fine tuning of the
calendar, or with the rotation of the moon so that every years the
phase of the moon correspond to different days). If we also
consider that the clocks can vary time periodicity through
exchange of energy (i.e. interaction), we see that the evolution
of a simple system of interacting periodic phenomena is in general
very chaotic [e.g. the orbits of three gravitational bodies].
Similar to an ordinary calendar or stopwatch the arrow of time in
the quantum world can be therefore described in terms of the
“ticks” of the internal clocks of the particles, and in
principle the external time axis can be dropped. This description
of reality terms of elementary cycles also provides a better
understanding of problems related to the notion of time in physics
[2,4,31]. In particular the de Broglie internal clock
associated to an elementary particle, as our ordinary clocks, can
be conventionally chosen to be clockwise or anticlockwise. Only
the reciprocal combinations of the ticks of these clocks are
important. However if invert a single clock with respect to the
others we obtain a different physical system. This corresponds to
pass from a particle to an antiparticle and vice versa.
The periodicity of the de Broglie internal clocks of the
elementary particles is tipically incredibly fast with respect to
our human time scale. In fact their periodicity is always
faster that the proper time periodicity, which is fixed by the
rest mass M of the particle T_0 = h / M c^2 - in fact the rest
energy is related to the mass (E=Mc^2). The proper time
periodicity is the is equal to the time that takes light to pass
through the Compton wavelength of a particle, which for the
electron is 10^-12 m. In this way it is possible to see that
the order of the intrinsic time periodicity of an electron
is 10^-21 s. To have an idea, the periodicity of the Cs-133
clock is, by definition, of the order of 10^-10 s. This
means that for every “tick” of the Cs-133 clock, an electron
(the lighter particle except neutrinos) does a huge number of
“ticks”, comparables with the age of the universe expressed in
solar years (i.e. it is like comparing a solar year with the age
of the universe). The proper time periodicity is the is equal to
the time that takes light to pass through the Compton wavelength
of a particle, which for the electron is 10^-12 m. The
heavier the mass, the faster the periodicity (the mass scale
explore by LHC corresponds to the time scale 10^-27 s). The modern
resolution in time, nearly 10^-17 s, is still far from these
scales. However the internal clock of the electron has been
indirectly observed in a recent interference experiment [38]. This
intrinsic periodicity allows a semi-classical formulation of the
spin and the intrinsic magnetic momentum of the electron as
already noticed in 1932 by Schrödinger in his
Zitterbewegung model. Remarkably in my papers I have rigorously
mathematically proven that the assumption of intrinsic periodicity
is sufficient to completely derive the fundamental quantum
behavior of elementary particles.
III. The harmony of elementary
particles
An elementary system
constrained to have intrinsic periodicity can be typically
represented as a string vibrating in compact dimensions.
Therefore, as shown in fig.3, we will represent elementary
particles as tiny vibrating strings. Their quantum behavior can be
understood in terms of harmonic vibrations along the space-time
dimensions [4].
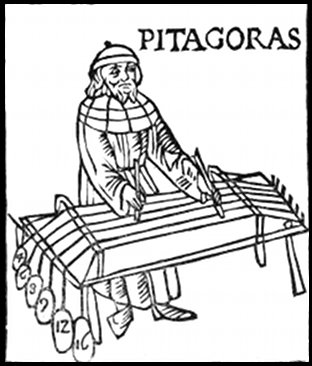 Fig.2
A
representation of Pitagoras playing strings. A vibrating string
can be considered one of the most fundamental physical systems
in nature. It is in fact at the base of many theories in
physics. The expansion in harmonics modes is also at the
origin of mathematics, as well as of the concept of harmony in
art.
|
As known since Pythagoras, see fig.3a, a
system (e.g. a string) constrained in a compact or periodic space
can only vibrate with discretized (quantized) frequencies.
That is a homogeneous string, which in our case corresponds to a
free particle, can be represented as a homogeneous string
vibrating with fundamental periodicity T=1 / v , so that the
resulting harmonic frequency spectrum is v_n = n v.
According to the de Broglie, the frequency v multiplied by the
Planck constant h (which can be imagined to be the “compression
coefficient” of the string) corresponds to an energy E = h v. As
a consequence of the assumption of intrinsic periodicity every
elementary isolated particle exhibits a harmonic quantized energy
spectrum E_n = n E = n h v. This is in perfect analogy with
the semi-classical quantization of a “particle in a box”
fid.3a.
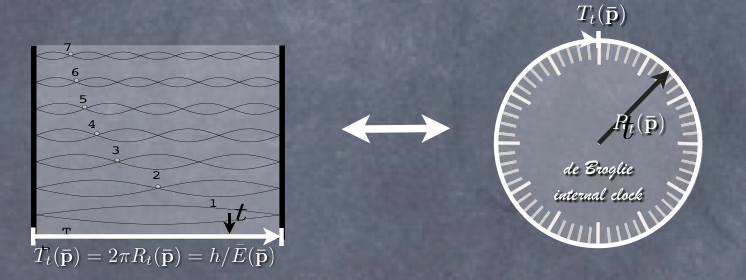 Fig.3
A
de Broglie “periodic phenomenon” describing an elementary
particle can be either imagined as a “de Broglie internal
clock” or as a vibrating string with de Broglie periodicity
T=1/v. As well know, a vibrating string can be expanded in
harmonics modes whose quantized harmonic spectrum v_n = n v is
fixed by its length. In this way it is easy to see that to the
intrinsic periodicity of an elementary particle there is
naturally associated a quantized energy spectrum E_n = n
E = n h v. It is possible to show that, analogously to the
quantization of a particle in a box, this se-classical
quantization reproduces the basics aspects of ordinary quantum
relativistic mechanics.
|
To understand the physical meaning of
this quantization we may consider the case of the Black-Body
radiation, which historically was one of the first arenas of QM.
In the so-called Black-Body the electromagnetic radiation is
continuously emitted and absorbed by the walls of a cavity kept at
fixed temperature. We must also notice that the photons are
massless particle, M=0, so that thy have infinite proper time
periodicity, i.e. the internal clocks of the photons are
frozen. This means that their time periodicity can span from
infinite to zero. Thus, for those components of the
electromagnetic radiation with long time periodicity the spectrum
can be approximated to a continuous. That is the effect of the
periodicity can be neglected like in a very long string that can
vibrate with all the possible frequencies, like a string
constrained to vibrate within very small dimension. On the other
hand, for those components with very small periodicities (the
component with very high frequencies are called UV component), the
effect of the periodicity cannot be neglected and the quantization
of the energy spectrum E_n = n E becomes evident. This is
nothing but the Planck description of the Black-Body radiation,
introduced to avoid the so called UV catastrophe. We can
imagine that for photons with small energy w.r.t. the thermal
noise their time periodicities is destroyed (decoherence), whereas
if the photons are sufficiently energetic their periodicity can
not be neglect and we can observe the quantization of the energy
spectrum.
At this point we may consider the
relativistic Doppler effect, so that the frequency, and thus the
periodicity, of a periodic phenomenon varies in a relativistic
way. If we consider the relativistic modulation of periodicity
associated to variations of reference frame it is easy to find out
that the energy spectrum of an intrinsically periodic phenomenon
is nothing but the energy spectrum prescribed in ordinary QFT by
the famous “second quantization”.
This is the first
element of a long chain of exact correspondences with ordinary
QFT. For instance, a vibrating string is the typical classical
system which can be described in a Hilbert space, the relativistic
wave describing our string (Klein-Gordon equation) is the “square”
of the Schrödinger equation, the evolution is Markovian and
unitary (i.e. it can be cut in infinitesimal evolutions), it is
possible to define an Hamiltonian and Momentum operator, and so
on. Remarkably, by integration by parts, from this intrinsic
cyclic behavior it is possible to derive the ordinary commutation
relations of QM as well as the Heisenberg uncertainty relation.
Intuitively, to establish with good accuracy the frequency Dv, or
equivalently the energy DE=h Dv of a periodic phenomenon it is
necessary to count its ticks for a very long time with respect to
its fundamental periodicity (i.e. to count a large number of
periods), according to the relation DEDt ≥ h.
Even the
most powerful mathematical tool of QFT, i.e. the Feynman Path
Integral (FPI), can be derived in a extremely intuitive way from
the assumption of intrinsic periodicity, [4,32]. The FPI
prescribes that the quantum evolution of a particle is affected by
self-interference of all its possible paths starting from A to B.
But in classical mechanics it is only possible a single “straight”
path between two point, so that in the ordinary formulation the
FPI can be only achieved by relaxing one of the pillars of
classical mechanics, the least action principle. But this is
not the case of a classical cyclic geometry. If we imagine
to drawn all the possible “straight” lines between two points
in a cylinder, see fig.4, we immediately see that an infinite set
of classical paths with different windings number are possible. In
few words a classical periodic phenomenon can self-interfere and,
as proven in [1-4], its classical evolution is naturally described
by the ordinary FPI of QM. From this also follows a similarly
natural interpretation of double slit experiment [32] [another
possible way to see the wave-particle duality in this formulation
is that an elementary system is in fact represented as a string
which is not a localized object, but if its time periodicity is
small it can be approximated to a particle. In fact in the
classical limit a massive string turns out to have nearly zero
time compactification and infinite spatial compactification. If we
also notice that such a string is mainly always localized inside
its Compton length, we find that in the classical limit such a
string is a point particle living in an infinite three dimensional
space and effective time evolution].
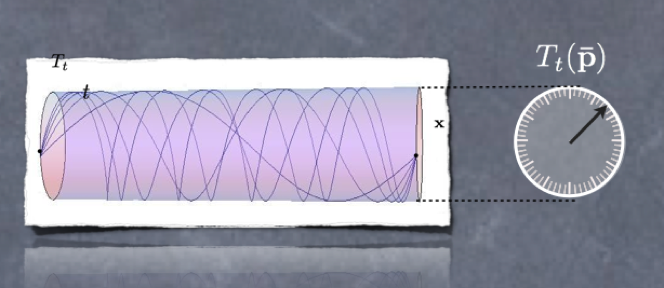 Fig.4
In
an intrinsically periodically phenomenon, such as that
associated to an elementary particle, the evolution from a give
initial configuration to a final configuration is given by the
interference of all the possible paths with different winding
number. It is possible to show that this sum over such
classical paths associated to a cylindrical geometry reproduce
the Feynman Path Integral.
|
Indeed, in this theory the
quantized energy spectrum of a particle is described by the
vibrations of a “periodic phenomenon” along the time
dimension. Similarly, the vibrations along the modulo of spatial
dimension and of the angular dimensions describe the quantization
of the momentum and of the angular momentum, respectively. It is
interesting to note that the theory is the full relativistic
generalization of sound theory. Sound theory, developed by the
Nobel laureate 1904 Reyleigh, is actually at the origin of the
modern quantum formalism. A sound source is an object vibrating
along spatial dimensions in a classical wave framework. A quantum
system turns out to be an object vibrating along space-time
dimensions in a relativistic wave framework [4]. The idea that
quantum mechanics could be related to space-time vibrational modes
has recently inspired the Nobel laureate F. Wilczek which has
recently published two papers on a similar idea that he named
“time-crystal”, [37] (a crystal is characterized by a periodic
structure, and it can be described by considering a single period,
see Brillouin zone).
Indeed this description also yields the Bohr-Sommerfeld
quantization which is historically was one of the first
quantization prescription. Its most famous application is in
the description of quantized orbits of the Hydrogen atom which can
be obtained by considering only closed orbits, i.e. a periodicity
conditions (modulo a twist factor) for the electrons orbitating
around the nucleus. It can be shown, for instance, that the
harmonics expansion of the temporal and angular vibrations of a
periodic phenomenon in a Coulomb potential gives the correct
atomic orbitals of the hydrogen (or helium) atom, [2-4] (similarly
it is possible to describes Zeeman effect ).
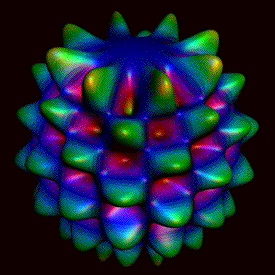
Fig.5a
Fig.5b
Fig.5c In the hydrogen atom the allowed
orbits are those in which the periodic phenomenon associated to
an electron does an integer number of de Broglie time periods,
i.e. closed orbits along the time direction (modulo twist
factors). The winding number along the time dimension describes
the principal quantum number. This is nothing that a
generalization of the original description given by Bohr,
Fig.5a. The azimuthal quantum number and the
magnetic quantum number can be obtained by considering the
quantization of the vibrational modes associated to the
spherical spatial periodicity of the orbits, Fig.5b. The
resulting description is therefore the generalization of the
vibrational modes of spherical membrane allowed to vibrate in
the time direction, fig.b.
|
IV.
An unexpected unified scenario
As shown with deep
mathematical detail in recent publications [1-4,8,9], the axioms
of ordinary QM can be inferred directly from the simple and
natural assumption of intrinsic periodicity of elementary systems.
This promotes intrinsic periodicity as the physical hypothesis
(the “missing link”) to complete the “incomplete revolution”
of QM, see also [39]. In fact intrinsic periodicity is also a way
out to the Bell’s theorem (it states that theory with
local-hidden variables cannot consistently reproduce ordinary QM).
In fact, the quantization condition in the theory is represented
by the assumption of intrinsic periodicity, as for the
semi-classical quantization of a particle in a box. This
also means the QM is retrieved without introducing any
local-hidden-variable in the theory (time is a physical variable
that can not be integrated out and the assumption of periodicity
is an element of non-locality in the theory). As noticed in [1],
if we try to reformulate the Bell’s theorem within our theory we
find again a formal correspondence with the inequalities of
ordinary QM. Therefore we may speak about determinism.
We
may thing in the following way. A typical periodic dynamics of a
typical quantum system (governed by the so-call quantum
electrodynamics) is so intrinsically fast (the intrinsic
periodicities associated to electrons are always faster than
10^-21 s) w.r.t. our resolution in time that at every observation
the system appears to be in an aleatoric phase of its cyclic
evolution. Intuitively, a phenomenon with such a fast
periodicity is like a dice (“de Broglie deterministic Dice”,
see [3,31]) rolling too fast w.r.t. our resolution in time, so
that its outcomes can only be described statistically. As already
noticed by G. ‘t Hooft, the statistical laws associated to a
periodic phenomena formally corresponds to the ordinary quantum
harmonic oscillator [35], which in turns is the basic ingredient
of ordinary QFT. According to this mathematical formulation, QM
can emerge as a statistical description of the intrinsically
deterministic periodic dynamics of such fast vibrating strings,
i.e. de Broglie dices. Thus an imaginary observer with
infinite time resolution would be able to resolve the
deterministic dynamics of the de Broglie deterministic dice and in
principle to predict the outcomes, as for an ordinary dice. That
is such an observer would not have fun playing dices.
This interpretation of QM not only is interesting for the advance
on the conceptual and philosophical knowledge of the quantum world
and the concept of time, it is also interesting to improve
computational methods and to address open questions in HEP. To see
this we must introduce interaction in our theory. Since
temporal-spatial periodicity and energy-momentum are two faces of
the same coin, we can equivalently describe the retarded and local
variations of energy-momentum occurring during relativistic
interactions in terms of corresponding modulations of
temporal-spatial periodicity of the elementary particles. In
particular we may note that Einstein derived his description of
gravitational interaction of general relativity by considering the
modulations of temporal-spatial periodicity associated to a
Newtonian gravitational potential. In general, local modulations
of periodicity of reference clocks can be equivalently encoded in
corresponding local deformations of the underlying space-time
metric. Fig.5, show the modulation of periodicity in
linearize gravity and the corresponding deformation of
the metric, a.k.a. Schwarzschild metric. As well known, reference
clocks in a gravitational well go slower w.r.t. those outside.
Therefore the assumption of intrinsic periodicity is fully
consistent with special and general relativity as long as
modulations are considered. In fact relativity sets the
differential structure of space-time without giving any particular
prescription about the boundary conditions. The intrinsic
periodicity of the elementary particle can be formalized through
periodic boundary conditions which are absolutely consistent with
th variational principle of relativistic theories.
The geometrodynamical description of gravity and its relation to
clock modulation is well known. As mathematically proven in
[1], by considering the undulatory nature of elementary particles,
the geometrodynamical description of gravitational interaction in
GR can be extended to gauge interactions. This represents an
important and conceptual step towards a unified geometrodynamical
description of all the fundamental interactions of nature and
constitutes an historical, exceptional further success of the
theory. Basically, a gauge field, through a formalism very similar
to the one of modulated signals, turns out to describe modulations
of periodicity associated to local transformation of reference
frame. This can be easily understood if we consider how an
electromagnetic field is generated by the trembling motion of a
charged particle in an antenna. Such a trembling motion can
be described as local transformation of reference frame of the
charged particle, and the resulting modulation of periodicity,
similarly to the Doppler effect, turns out to be described by the
Maxwell equations. In fig.6 this is shown together with the
corresponding local “rotation” of reference frame (e.g.
zitterbewegung). Remarkably, gauge symmetries, which in ordinary
QFT are internal symmetries whose existence must be postulated,
turn out to be related to space-time symmetries through the
assumption of intrinsic periodicity, and the Equivalence Principle
of General Relativity can be extended to gauge interaction.
Remarkably this was Weyl’s original proposal when he introduced
the idea of gauge invariance, but similar attempts can also be
found in Kaluza’s and Wheeler’s works. Moreover,
when the modulation of periodicity of all the harmonic modes is
considered, the classical evolution of our vibrating string turns
out to be described by ordinary quantum electrodynamics (QED).
This means the quantum behavior of gauge interactions can be
derived directly from cyclic dynamics, so that, in principle,
Feynman diagrams can be expanded in harmonics and calculated
semi-classically. That is, the explicit quantized spectrum of a
periodic phenomenon could regularize the infinities of the loop
diagrams (this possibility is also confirmed by
Light-Front-Quantization, twistor theory, holography, AdS/CFT; all
these theories have important formal and conceptual analogies with
the theory described here).
Fig.6a
fig.6b
Fig.6c
Fig.6b
describes the ordinary relativistic description of
gravitational interaction in terms of space-time deformation or
equivalently in terms of modulation of space-time periodicity
of reference clocks which therefore run slower, i.e. are
redshifted, with respect to clocks outside the gravitational
well. Under the assumption of intrinsic periodicity the same
geometrodynamical description can be applied to the other
fundamental interactions in nature. In fig.6a shows the
modulation of periodicity of a de Broglie internal clock
interacting electromagnetically together with the corresponding
local polarized rotation of space-time metric [1]. In fig.6c it
is represented the logarithmic freeze-out of the
quark-gluon-plasma which, analogously to the Newton’s law of
cooling, passes logarithmically from an hot regime
characterized by small periodicities to a cold regime with long
time periodicity. The corresponding deformation of space-time
is a warped metric and resulting dynamics are those prescribed
by ordinary quantum chromodynamics [4,32].
|
Periodicity conditions impose that the
gauge field can vary only by finite amounts in unit h/e (being e
the electric charge). Thus the magnetic flux is quantized and the
electric current can not vary continuously. Therefore the theory
explain superconductivity in a immediate and material-independent
way. This also means that intrinsic periodicity points towards a
gauge symmetry breaking condition alternative to the Higgs
mechanism. According to [40], from these quantization condition of
the magnetic flux (a.k.a. Dirac quantization) it is possible to
derive realistic models for the electroweak symmetry breaking in
HEP. Another relevant result is that, according to the Bjorken
Hydrodynamical model [42], in an accelerator experiment the energy
of the fields constituting the Quark-Gluon-Plasma (QGP) decay
exponentially during the freeze-out. In thermal QCD this
corresponds to the Newton Law of Cooling, in fact the QGP can be
represented as a volume of hot fluid. Therefore, as shown in
Fig.7, the periodicity of the fields has an exponential
modulation. As shown in my recent e-print [30], this can
consistently describe the asymptotic freedom of
Quantum-Chromo-Dynamics (the third gauge interaction). Least
but not last, form the formalism of extra-dimensional theory it is
possible to note that the extra-dimension acts in surprising
mathematical analogy with the intrinsic proper time periodicity of
a de Broglie internal clock. This indicates a correspondence
between periodic phenomenon and extra-dimensional theory [30],
similarly to Klein’s original proposal, and justifies the good
behavior of extra-dimensional theory without introducing an
(unobserved) extra-dimension. Considering this dualism, the theory
also provides an elegant explanation for the quantum to classical
correspondence of at the base the AdS/CFT correspondence, which is
one of the greatest open questions of the last decade of
theoretical physics [42]. The quantization of elementary particles
trough the assumption of intrinsic periodicity has important
applications in condensed matter where actually semi-classical are
widely used. In [33] we have for instance pointed out that such an
approach can be used for a formulation of superconductivity based
on fundamental principle of QM mechanics rather than on
microscopical properties of the material. Similarly in [33] we
have applied the same idea to interpret the behavior of electron
in graphene and carbon-nanotubes, or to describe laser and
Black-Body radiations. This just to mention few potential aspects
explored in this research project. But for the general
nature of the assumption of intrinsic periodicity the applications
of this method can go from cosmology (see cyclic model of the
universe, or quasi normal modes in black-holes) [31,32] or to
quantum gravity [1,31], to biology or econophysics (see cyclic
models).
V.
The origin of masses
Such a theory potentially opens the
way to new challenging new scenarios in phenomenological aspects
of high energy physics. For instance, by working in analogy
with current extra-dimensional Higgsless/composite-Higgs models
[9,10] it can be used to understand the fundamental nature of the
electroweak symmetry breaking mechanisms in analogies. Roughlty
speaking this means to investigate the problem of the origin of
the masses in the more fundamental and original approach allowed
by my theory. We have seen that the masses of elementary particles
have precise geometrical interpretation in terms of proper time
periodicity of the elementary particles, i.e. in terms of the
compactification lengths of the vibrating strings [1-4,30,31]. The
question of the origin of the masses that LHC is trying to solve
can be therefore reformulated in the following way: why is
nature playing that particular string chord? In this theory such a
problem can be addressed by using the analogies with
superconductivity [1,32] and the Dirac string description of
monopoles [40]. Another possibility allowed by the theory is a
semi-classical computation of loop diagrams in QED in terms of the
harmonics of a vibrating string. The feasibility of this
point is indicated by the recent successes of similar recent
calculations in empirical integrable models, e.g.
Light-Front-Quantization [44], Twistors, AdS/CFT, Holography
[42].
With the publication of [1-4],
the first critical, foundational stage of this research project
can be considered successfully accomplished. As in a Pandora box,
the simple assumption of intrinsic periodicity, if well
implemented, has showed to unlock most of the historical (and
sometimes forgotten) open questions of modern physics in an
extremely natural way and with an amazing mathematical beauty.
Now it is time for brave physicists to really face challenging,
feasible, natural issues of nature.
References
[1] Gauge
Interaction as Periodicity Modulation. Donatello Dolce, (Melbourne
U., CoEPP). Nov 2011. 53pp. Published in Annals of Physics, 327
(6) June 2012, pp. 1562-1592, DOI: 10.1016/j.aop.2012.02.007
[2]
On the Intrinsically Cyclic Nature of Space-Time in Elementary
Particles. Donatello Dolce, (Melbourne U., CoEPP). Sep 2011. 13pp.
Published in J. Phys.: Conf. Ser. 343 012031, 2012.
[3]
de Broglie Deterministic Dice and emerging Relativistic Quantum
Mechanics. Donatello Dolce, (Melbourne U., CoEPP). Jan 2011. 10pp.
Published in J. Phys.: Conf. Ser. 306 012049, 2011.
[4]
Compact Time and Determinism for Bosons: foundations. Donatello
Dolce, (Mainz U., THEP) . MZ-TH-09-06, 2010. 43pp. Published in
Found.Phys. 41:178-203,2011.
[5]
Holographic approach to Higgsless models. D. Dolce (Universitat
Autonoma de Barcelona and IFAE),R. Casal- buoni, S. De Curtis, D.
Dominici (Florence U. and INFN, Florence) . Feb 2007. 23pp.
Published in JHEP 0708:053,2007
[6]
Playing with fermion couplings in Higgsless models. R. Casalbuoni,
S. De Curtis, D. Dolce, D. Dominici (Florence U. and INFN,
Florence) . Feb 2005. 20pp. Published in
Phys.Rev.D71:075015,2005,
[7]
The Scalar sector in an extended electroweak gauge symmetry model.
Stefania De Curtis (INFN, Florence U.) , Donatello Dolce (Florence
U.) , Daniele Dominici (INFN, Florence U.) . Jul 2003.
12pp.
Published
in Phys.Lett.B 579:140-148,2004
[8]
Deterministic Quantization by Dynamical Boundary
Conditions.
Donatello
Dolce, (Mainz U., THEP) . MZ-TH-10-24, Jun 2010. 4pp. Published in
AIP Conf.Proc.1246:178-181,2010.
[9]
Quantum Mechanics from Periodic Dynamics: The Bosonic
Case.
Donatello
Dolce, (Mainz U., THEP) . MZ-TH-09-23, Jan 2010. 6pp. Published in
AIP Conf.Proc.1232:222-227,2010.
[30]
AdS/CFT interpretation of a Virtual Extra Dimension. Donatello
Dolce, (Melbourne U., CoEPP). Mov 2012. 40pp. Submitted to Annals
of Physics e-Print: arXiv:1110.0316
[31]
Clockwork Quantum Universe. Donatello Dolce, (Melbourne U.,
CoEPP). Feb 2011. 8pp. Forth Price, FQXi contest 2011 “Is
reality Digital or Analog?” web page:
http://www.fqxi.org/community/essay/winners/2011.1#dolce
[32]
Compact Time and Determinism for Bosons Donatello Dolce,
(Melbourne U., CoEPP). Mar 2009. e-Print: arXiv:0903.3680v1 -
arXiv:0903.3680v4.
[33]
Gauge Symmetry Breaking without the VEV and other considerations
about superconductivity Donatello Dolce, (Melbourne U., CoEPP).
Feb 2012. 16pp. Submitted to Annals of Physics
[34]Flavor
Physics in the Randall-Sundrum Model M. Neubert, et. al. JHEP
0810:094,2008.
[35]Determinism
in free bosons. G. ‘t Hooft,
Int.J.Theor.Phys.42:355-361,2003.
[36]How
a wave function can collapse without violating Schroedinger's
equation, G. ‘t Hooft, arXiv:1112.1811
[37]Quantum
Time Crystals,F. Wilczek,arXiv:1202.1523;Classical Time
Crystals,F. Wilczek,e.l.arXiv:1202.2537
[38]
Search for the de Broglie Particle Internal Clock by Means of
Electron Channeling, P. Catillon, et.al,
Found.Phys.38(2008)659.
[39]
A missing link: What is behind de Broglie’s “periodic
phenomenon”? R. Ferber, Found. Phys. Lett., 9, 6,
(1996),575–586.
[40]
Electroweak Symmetry Breaking From Monopole Condensation C. Csaki,
Y. Shirman, J. Terning, Phys.Rev.Lett.106:041802,2011
[41]
Non-equilibrated post freeze out distributions, V. K. Magas,
et.al, Eur. Phys. J. C30 (2003) 255–261.
[42]
Anti-de Sitter space, thermal phase transition, and confinement in
gauge theories, E. Witten, Adv. Theor. Math.
Phys.2(1998)505
[43]
Gravitation and electricity, H. Weyl, Sitz. Preuss. Akad.
Wiss. Berlin (Math. Phys.) 1918 (1918) 465.
[44]
Electron in a transverse harmonic potential, H. Honkanen,
PoS LC 2010 (2010) 004.
|